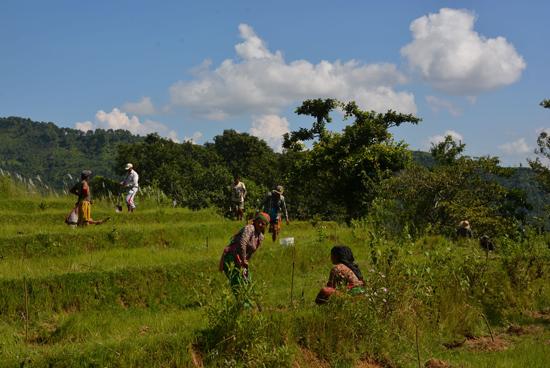
On the steep hills at the foot of the Himalayas, Nepalese people practiced organic agriculture for more than 2,500 years. By hand they terraced slopes and channeled water. Even fierce summer rains did not cause strong erosion across the cultivated terraces. Oxen plowed the tiny terraced fields two, often three times a year. Their dung, along with that of cows, buffalos and goats, acted as fertilizer. The nutrient cycle was closed, and the fertility of the ground was preserved for over two and a half millennia. Though their work was hard and life expectancies often low, these farmers rarely suffered from hunger.
Now globalization with its radios, TV, cell phones, motorcycles, buses and market access has reached even the most remote mountain villages and awakened an understandable desire for consumer goods, medicines, confectionary, gas cookers, and a modicum of comfort. These things do not spring forth from the earth but are produced by machines far away. The rural farmers cannot wrest the income necessary to satisfy their desires for these new things from the fields that they painstakingly tilled. This has led to villages becoming depopulated, especially of the men and young school graduates, who move to the cities or join the hordes of guest workers in Arabic countries. Though they may lose their sense of identity and dignity, their daily wages hold more purchasing power than the grain and vegetables grown on their fields at home.
Back in the villages, it is common that only the elderly, women, children, and the dispirited remain. Workers are sorely missed. The thousand-year-old terraces are falling into ruin; uncultivated ground cracks, walls crumble, erosion washes away topsoil, and wells dry up. Those who remain only cultivate the gardens around their houses, feed the goats from what remains on the withering fields, and live on the little the husbands and sons send to them from afar. They feel abandoned by fortune and are often filled with self-contempt for having been too weak to get away.
Fig. 1: Fallow terraces at 1,200 m above sea level in Ratanpur (Tanahu), an over six-hour drive west of Kathmandu
At the same time, the country’s balance of foreign trade suffers more and more losses. The industrial nations, with their machines and more efficient labor organization, can produce everything far cheaper than Nepal. The few export products that the country could manufacture without expensive machines would generate too little income to support workers. Nepal therefore imports not only machines, cars, gas and chemical fertilizer from India, but also rice, flour, vegetables, fruits and even eggs, all items that could be produced in this subtropical climate with ease.
Foreign aid can do little against these social and economic distortions. From the inside, there are no easy and quick solutions that the political caste might grasp. However, where chaos manifests, there is also opportunity. The land around the villages that has been abandoned lies uncultivated beneath the sun and rain, beckoning new development.
Increase of Local Value
In the southern lowlands of Nepal bordering India, where fertilizer, pesticides and machines are cheaper and where the market access is easy, the cultivated soils are highly degraded, as they are in large parts of India, and the groundwater is frequently tainted with toxins, especially nitrate.
By contrast, in the poorly accessible hills and deep in the mountains of Nepal where no fertilizers and pesticides can be purchased, most of the soils are still fertile. The sun in the northern subtropics is intense and temperatures never fall below freezing, yet it is rarely too hot either. Fields are continuously amended with compost, and with a yearly average rainfall of 2,000 mm there is enough water available to provide irrigation through skillful distribution even in the dry months. These are suitable conditions for most crops to grow in abundance. Yet on the steep terraces, it is not profitable to grow grain beyond that needed for personal nutrition. The villages are too far away from the marketplaces, the roads are bad, it is nearly impossible to mechanize production, and there are labor shortages as well.
All of these impediments can be overcome if higher-value crops are planted and processed on-site. With local value addition, durability of the goods increases, the transport volume decreases, and marketing becomes economically viable. In this manner, silk from mulberry leaves can be produced and sold instead of rice; essential cinnamon oil instead of corn; nuts instead of potatoes; and dried banana or mango chips instead of millet.
Fig. 2: Many villagers take part in the communal planting of the forest gardens.
Basic foods for the families should continue to be grown on-site, and the gardens surrounding the houses usually suffice for that. However, in order to provide families with a regular income, the uncultivated land must be utilized in a manner that creates more value than traditional farming.
Cultivation of Forest Gardens
Forest gardens have the advantage over traditional crops that less effort is required for their cultivation. Such gardens would provide the potential for the remaining women and elderly of the village to return fallow fields to cultivation. The amount of work required can be adjusted to suit the villagers’ physical capacities and motivation. Over time the outcome and success can be used to re-attract the lost sons and fathers.
For the most part, trees grow on their own once they have firmly taken root. After this point, there is little need for fertilizing, crop protection, ploughing, weed removal, and thus only the harvest has to be organized and occasional pruning. However, even in the tropics it generally takes three to five years for the trees in a forest garden to be ready for the first harvest. This presents the prospect of considerable initial work without any short-term financial return of the investment. To bridge this income gap, secondary crops such as ginger, turmeric, lentils or onions can be grown during these initial years, however, these crops may require extensive effort, which is challenging where labor is scarce.
Fig. 2: Black lentils intercropped in a one-year-old forest garden with bananas, cinnamon and Paulownia.
There are several human and financial costs connected to the creation of forest gardens. The fallow, partly overgrown land must be prepared and saplings bought and transported or raised onsite. Planting holes need to be dug and amended with compost and biochar, which have to be produced and brought to the location. The trees must be planted with care, mulched and watered on a regular basis. The soil around the trees needs to be diked and drained during the rainy season, and tree basins have to be dug during the dry season to hold the irrigation water long enough to infiltrate deeply into the root zone.
One of the main problems is that during the particularly dry periods from February to April there is hardly any water available, and it needs to be carried laboriously from faraway wells. To secure the survival of not just a few but hundreds of trees, the timely construction of water retention pits and ponds and the buying of irrigation hoses are crucial. Subsequently, the trees must be protected not only from browsing by game but also from the goats of the often-envious neighbors. Importantly, the individual planting steps and cultivation tasks throughout the year have to be meticulously and proactively organized, delegated, and carried out in time, otherwise all of the work will have been in vain and the trees will die. This focus on correct and timely action frequently proves to be the most difficult task to achieve. The successful establishment of forest gardens is thus mainly a social challenge rather than a simple transfer of agricultural know-how.
All in all, the cultivation of forest gardens calls for a serious effort. Investments need to be made. Planning and motivation are required. A return only starts coming in after three to four years, and only provided that everything goes according to plan: that no storms wash away the labor’s results, no fires break out, no neighbors sabotage the garden, no animals devour the month-long labor in one short day, no plague of locusts descends and, most importantly, that all necessary tasks are carried out at the right moment.
These are a lot of uncertainties and risks when farm families can barely meet their basic needs and desires, let alone raise the necessary capital for seeds, water retention pits and irrigation hoses. For thousands of years it has been common in the Himalayas to leave the fate of fortune and prosperity in the hands of religion and be satisfied with dreaming, rather than to risk struggling in vain. The problem is the same in Nepal, India, Madagascar, Haiti, Andalusia, Ethiopia, Iran, the Greek Isles and many other countries where agriculture is a mainstay for a high percentage of the population. It partly explains why, to this day, there are more trees being cut down than planted, why the fertility of the soil is decreasing, and why people still suffer from the lowest food diversity in places where anything would grow.
Vegetal Carbon as Income and Commodity
There is a way that could make the investments and efforts of the first three years viable for every farming family – not through subsidies or donations, but by connecting farmers to the international carbon market. The planted trees not only promise the profits from their fruits, seeds, leaves, woods, oils, resins and medicine, but they also absorb CO2 from the atmosphere and store the carbon within their wood, leaves, and roots, and also in the soil.
Fig. 3: Mulberry tree plantation six months after planting.
With the increased pressure to limit climate change to a safe level of 1.5°C, as has been agreed at the Paris COP21 conference, worldwide management of carbon is vital. The equilibrium temperature that will result from climate change is directly proportional to the concentration of greenhouse gases (GHG) such as CO2 and methane (CH4) in the atmosphere. Even as humanity strives to reduce CO2 emissions over coming decades, the CO2 concentration will continue to increase. It takes a century to decay atmospheric CO2 to 50%, while 20% of it remains for millennia (Hansen et al., 2016). Because of this the amount of warming due to CO2 by each nation, is closely proportional to the cumulative amount of CO2 emitted, shown for selected nations in Figure 6.
It is a common agreement that the current CO2 concentration in the atmosphere, along with the unavoidable future emissions, commits the planet to warming of more than 2oC over preindustrial average temperature (Rockström et al., 2016). Sustained warming above 1.5oC risks triggering uncontrollable feedback effects and runaway climate change. To keep climate change within “control”, we not only have to reduce emissions quickly, but we also must actively withdraw the excess CO2 from the atmosphere over coming decades (Boysen et al., 2016; Rockström et al., 2009, 2016, 2017, Hansen et al, 2016). If the excess C can be withdrawn and sequestered for at least 100 years, preferably longer, this would allow for the level in the atmosphere to decline enough to bring warming back within safer bounds.
At present, only photosynthesis by plants and algae can a draw down substantial atmospheric CO2. To decrease the atmosphere’s carbon content, it is thus necessary to increase the worldwide capacity of net primary productivity and the amount of carbon stored away in biomass, in the ground and in products made from plant material. We need more plants, more trees, more carbon-accumulating soil and better strategies for utilizing biomass into long-term C storage.
Whether a tree grows in Nepal, Guinea, Mauritania or Canada, each ton of carbon that the tree extracts from the atmosphere is a service to the global climate. The price for such a service should therefore be the same worldwide. The wealthy nations most responsible for climate change can redeem their debt in part by assisting, financially and with technology, the undeveloped nations to increase biomass growth and carbon sequestration. Especially in the tropics and subtropics, net primary productivity is high, land and forests are in dire need of remediation, and the labor is available and hungering for equity.
Towards an Ecological Future with a Uniform Price for CO2
Every tree stores in its trunk, roots and branches a certain amount of carbon that has been extracted as CO2 from the atmosphere. The stored carbon within a particular tree can be calculated from the volumes of the trunks, branches and main roots and their material densities, using an average carbon content of 50% by weight (Toensmeier, 2016).
A catalog of tree species including every tree’s average annual increase in height, trunk diameter and volume as well as carbon uptake of the trunk, branches and roots was initially assembled from literature references. The precision can be improved with measurements taken from forest cultivation projects, thus providing specificity to location, soil type, climate, altitude, exposure and form of intercropping. As the data accumulates year after year, the calculations will accordingly become increasingly accurate and adapted to the location.
Fig. 4: Aerial picture of a 2.5 years mixed tree plantation on an abandoned rice terrace in Ratanpur.
Currently, the catalog of tree species features 58 species and varieties where the average growth and C-accumulation rates are known in the given climate conditions. When farmer families in Nepal plant 600 trees from this catalog on one hectare of abandoned rice terraces we can calculate how much carbon is effectively pulled from the atmosphere as CO2 over the first 10 years, 25 or 100 years. As long as it can be guaranteed that the C accumulated in the forest garden is preserved and does not escape back to the atmosphere, this yearly average of carbon accumulation could be traded on the international market in the form of a CO2 certificate.
Basis of the carbon subscription
During the first 10 years, a mulberry tree extracts on average 33 kg of CO2 from the atmosphere, a cinnamon tree 90 kg, a Michelia champaka 233 kg and a frequently coppiced Moringa tree 450 kg. A bio-diverse mix of 600 fruit, nut, fodder, oil and timber producing trees per hectare results in 22 t C (81 t CO2) being pulled from the atmosphere over the course of the first 10 years.
We will use a German citizen as an example of a potential buyer of carbon credits, however, the example can be generalized from the data in Figures 5 and 6. On average a German citizen is responsible for the yearly emission of 11.5 tons of CO2 (Fig. 5), or 115 tons over 10 years, equivalent to the C accumulated in 10 years in a 1.4 ha forest garden in Nepal with 850 mixed trees. At 35 euros for one ton of CO2, it would cost 400 euros per person per year to achieve a climate-neutral lifestyle in Germany, and this will enable the cultivation and adequate care of corresponding tree gardens in Nepal. The Nepali farmer would not receive a subsidy or donation but a fair price for carbon services they offer on the market.
A German citizen could start to become climate-neutral, and climate responsible, for a monthly CO2 subscription of 33 euros, or a little over 1 euro per day. Even for someone earning minimum wage in Germany (8.84 euros/hour), this represents 3.7 hours per month, or a mere 10 minutes of work per day. For a lawyer earning 200 euros/hour the cost of climate neutrality equates to just 10 minutes of pro bono work a month, or less than 30 seconds a day. With 30 seconds to 8 minutes of work per day per resident, Germany could offset its current emissions and help to make the climate safe again. CO2 emissions from children, unemployed, invalids and pensioners could be covered by part of the inheritance tax.
The full measure of responsibility for an individual includes their entire legacy of GHG emissions caused during their lifetime, along with emissions imbedded in the infrastructure and lifestyle they inherited. The carbon of those legacy emissions lingers in the atmosphere and the ocean, causing climate change and ocean acidification. All past and future carbon emissions will have to be compensated. However, to start living climate neutral is the first decisive step, which, accompanied by ambitious measures to reduce future emissions, may in the long-term help to rebalance the historical carbon debt.
Fig. 5: Per capita carbon dioxide emissions for selected countries for (a) the year 2016, and (b) Cumulative for the period 1751 to 2016, i.e. the historical burden of the respective country. The emissions include those of agriculture and aviation, but not those caused by the production of goods in foreign countries. Compensating negative emissions through replanting of forests (LULUCF) are not included either. Source: www.columbia.edu/~mhs119/YoungPeople
How long does C remain stored in the forest garden?
The intention of the Ithaka program is to make the forest gardens so productive and adapted to the subtropical mountain conditions that farmers will have no reason to cut down the trees, burn the land or abandon this form of sustainable land management. Doing so would not only mean forfeiting a regular and stable income from the highly diverse and robust forest garden ecosystem, but also the food and fuel security for their family. In the face of climate change, which increasingly threatens arable crops with weather extremes, forest gardens are one of the most climate resilient agro-systems and the only natural system that guarantees year-round harvests. Once established, forest gardens are not labor intensive, and can be designed to be maintained by the remaining women and elderly people. Besides the interest the farming family would have in maintaining the garden in order to not lose their livelihood, we expect that with the increasing awareness of climate change and improving education, human reason will prevail to the extent that cutting forests will become unimaginable.
However, we do not want to sell the future but rather to conservatively prepare conditions for a productive future, with equitable contribution by the investor in C credits and a rewarding life and livelihood for farming families doing the work. To achieve this goal, we consider that a ton of CO2, extracted from the atmosphere and stored in the terrestrial system, can be sold as carbon credit and is worth 35 Euro. Based on this price, we help farmers to establish a forest garden that will within the next ten years accumulate in its biomass the amount of carbon corresponding to the subscription in carbon credits. However, Ithaka Institute advances and invests the full amount of the 10-years carbon subscription commitment during the first three years to cover the cost for the establishment and maintenance of the forest garden system and to compensate the farming families during these first unproductive years where the forest garden does not generate any family income. We expect that the farmers will continue to maintain, and will not cut down, trees for which they have vigorously cared over 3 years, and which will yield their most bountiful harvest after 10 years.
Although carbon credits are only sold for the first ten years of the forest garden’s life, the trees will accumulate more and more carbon beyond the first decade. The whole forest garden system is perennial and made to last. Some of the fruit trees may have to be replaced after relatively short periods (20 - 50 years) and larger shading timber trees may be cut for material uses (30 – 80+ years) and also replaced. However, to provide a conservative carbon accounting and guarantee climate relevant carbon -capture and bio-storage, we will sell only the forest garden carbon accumulated during the first 10 years. The subsequent carbon drawdown over future decades is part of the safety margin that some of the forest gardens will not last for a climate relevant time period, which we consider to be at least 100 years, and hopefully much longer (given that humanity recognizes in the next decades that indigenous forests or forest gardens will be maintained in perpetuity). Even if only 10% of the established forest gardens would have a lifespan of more than 100 years, more carbon would be stored in the long-term in this 10% than the originally certified amount. That means buying the carbon credits for the carbon accumulated over the first ten years buys most probably the bio-storage of a lot more not certified carbon.
The mutual responsibility for Forest Gardens
A carbon subscription has to be a long-term commitment for both the farmers who draw down the carbon from the atmosphere and the subscribers emitting excessive amounts of CO2. As the farmers have to guarantee to maintain the forest for at least ten years, the subscribers have to guarantee to pay for the trees that extract their carbon from the atmosphere for at least ten years. For both we expect that they will continue their commitment: The farmers for their forest gardens that provide them food, feed, fuel, and climate resilient preservation of their land; and the CO2-emitting subscribers who wish to take responsibility to at least neutralize their current share of national GHG emissions. After ten years, the carbon storage of each subscriber’s 850 trees (115 t CO2) reaches its certifiable limit, and if a subscriber wants to continue offsetting his/her emissions, 850 new trees will be planted elsewhere. This starts a new contract between farmers and subscriber to plant more forest gardens, supporting the livelihood of more farming families and their region.
While food production for 8 billion humans requires on an average 0.5 ha per person, the surface needed for the carbon sequestration of an European would be 1.4 ha every ten years. This makes clear how diligently the subscriber, and everyone in the high CO2-emitting nations, must work to rapidly reduce their national emissions. To achieve a sane and positive outcome for the planet mutually reinforcing actions are required: reducing emissions to zero; supporting equitable sharing of planetary resources (so that families everywhere can aspire to education, smaller families and healthy natural environments); drawing down and storing excess CO2 from the atmosphere; and fostering sustainable and resilient food production systems.
As mentioned, to be useful for the climate, a considerable part of the carbon that has been accumulated by trees must remain bound for time spans well exceeding 100 years. To demonstrate how this can be achieved we consider how the ecosystem processes the absorbed carbon and how farmers may utilize it. Figure 7 depicts the C flows in a hectare of forest.
Fig. 6: Estimated annual total C flows in tonne/ha/yr in (a) a tropical rainforest (Amazonia) and (b) a temperate deciduous oak-hickory forest (Tennessee, US). Gp = Gross photosynthesis; Rt = Total respiration; Ra = Autotrophic respiration; Rh = Heterotrophic respiration; Rl = Leaf respiration; Rw = Above ground wood respiration; Rr = Root respiration; Dag = Above ground litterfall & mortality; Dbg = Below ground detritus & exudation; T = C transfer below ground; ∆ag = Above ground biomass growth; ∆bg = Below ground biomass growth; ∆SOM = SOM matter growth. Source (numbers rounded): Malhi, Baldocchi & Jarvis. Plant, Cell and Environment (1999), 22, 715
Even though the the gross photosynthesis, Gp, is almost double in a tropical rain forest vs. a temperate deciduous forest, a much bigger percentage is retained as soil organic matter (SOM) in the temperate forest, resulting in the net annual C storage in above and below ground biomass and SOM being about 6t/ha/y for both forests.
Branches and Leaves
Most of the trees in the forest garden will be trimmed annually. The leafy branches are commonly used as animal fodder, while the woody remains are pyrolyzed into biochar. During digestion and metabolism, animals emit a portion of the carbon in the feed as CO2 and also some as methane (which on the short timescale now of concern for climate safety has ~80 times higher greenhouse gas potential than CO2). The remainder of the feed-carbon is later returned to the soil in the form of manure or compost. Nearly all of the carbonaceous molecules in the manure or compost are broken down by microorganisms over the next two or three years and released to the atmosphere, mainly as CO2 but partially as methane (e.g. when applied to rice fields). None of the feed carbon is added to the longer-term C-balance, though a portion of it supports the increase of soil organic matter (see below).
Pyrolysis of the woody remains of fodder branches and trimmings leads to 25 - 50% of the biomass carbon being transformed into biochar; the actual percentage depends on the technology and production parameters. If not all harvested leaves are needed for fodder purposes, the leaves can also be pyrolysed. Biochar can be used as a soil amendment to enhance the quantity and quality of biomass and food production. About 80% of the biochar carbon applied to the soil is stable for a period of several centuries at least (Lehmann et al., 2015; Zimmerman & Gao, 2013).
Trunk Wood
Depending on the kind of tree, trunk wood can be used for the construction of buildings or furniture. The carbon remains in place and stored for the life of the furniture or house, which will likely amount to an average of 35 to 50 years. Subsequently, at least 60% of the wood can be pyrolysed to biochar with a carbon retention efficiency of 25-50%. In this way 15 to 30% of the carbon that had been previously stored within the buildings and furniture can be sequestered in the ground as soil improvement for several centuries. The sawdust, wood scraps and other wood wastes like bark that are residues of furniture manufacture can be used for cooking in pyrolytic cookers (TLUDs), which also produce charcoal/biochar as a by-product. (Normally the C in timber used for containers or pallets has a much shorter circulation time back to the atmosphere, however in a carbon-managed world, the leftovers from these products could also be pyrolyzed for energy and charcoal after the “use by date.”)
Roots
Tree roots (and some of the trunk) usually remain in the ground and rot away slowly, the absorbed carbon being broken down and released back into the atmosphere over the course of 30 to 50 years. These roots are a major source for soil organic matter. This material could also partly be pyrolyzed.
Soil
As the forest garden matures the soil presents potentially the largest store of carbon. A 1% increase of humus content in the top 30 cm alone stores over 80 tons of CO2eq per hectare. If a forest garden is created on a soil lacking in humus, the carbon content in the ground can be increased by 4 to 7% with leaf mulch, decaying roots and branches, root exudation, and microorganisms, aided by the use of biochar-based fertilizers along with careful cultivation in the understory of the trees. The increase of humus content in the tropics depends on, and can be limited by soil type and climate, but can be enhanced by incorporation of nutrient boosted biochar substrates (creating Terra Preta like soils). It is difficult to provide reliable estimates, therefore every 5 years humus content will be measured, and we expect to integrate the data into the calculation of the C-balance after 10 years. However, for now, the additional carbon sequestration related to humus improvement is outside of the carbon-trading scheme.
Carbon Residence Time within the Carbon Cycle
Whether wood is processed into paper, used to build houses, or carbonized; whether the leaves are eaten by animals or fall to the ground as mulch; whether the roots rot in the ground or are excavated, burned or pyrolyzed, all carbon stored by or from a forest garden is eventually released into the atmosphere as CO2, and taken up anew by plants or algae. This is the natural carbon cycle in which plants functions as both a carbon and energy battery, chemically recharged by sunlight, and discharged again by metabolism or fire.
For the forest garden cultivation program to be effectively and continuously C-negative, i.e. contributing to C withdrawal from the atmosphere, the new agro-forest must store more C than the land use system it replaced (the baseline). If a natural forest were burned down to establish a forest garden, more carbon would be released than can be re-captured by the forest garden in many years to come. But if a degraded rice field is transformed into a forest garden, all biomass that is growing anew increases the carbon stock of the garden compared to the fallow-field situation before.
To make the cultivation system the most carbon-efficient, we must think beyond the planting of trees and boosting global photosynthesis capacity in order to increase the active extraction of CO2 from the atmosphere. Attention must be paid to prolonging terrestrial carbon residence times. We have to shift the overall carbon balance from carbon emissions (fuel combustion and biological respiration) to carbon extraction from the atmosphere (biomass production) and sequestration, so that the carbon stored in the terrestrial system (in biomass, soil, water and materials in human use) increases and the carbon in the atmosphere decreases to levels of ~ 350 ppm, which is expected to keep global warming at less than 1.5°. The main objective is thus to relocate atmospheric carbon to the terrestrial system for the long term. For this to be achieved, it needs on the one hand more plants that extract more carbon from the atmosphere, and on the other hand, viable strategies to maintain the carbon initially accumulated by plants for much longer times in the terrestrial system (more long-living trees, more black soils, more bio materials, more biochar.
Carbon absorbed by plants cannot be locked away (sequestered) forever. Even charcoal is broken down by microbes eventually or burnt after hundreds or thousands of years and return to the carbon cycle. Carbon does not settle; it moves at various paces between air, water, ground and biomass. However, employing suitable strategies can shift the balance between these four pools and increase the dwell time in terrestrial C pools. Reforesting degraded areas, increasing the humus content of agricultural land, or using wood and charcoal for building materials instead of burning them, shifts the carbon pool away from the atmosphere and towards terrestrial systems.
As an example, if a tree is converted to a wooden beam to construct a roof ridge, the carbon can be stored in the woodwork for the next three to four hundred years, until it has been decomposed by worms, fungi and bacteria and has been returned as CO2 back to the atmosphere. While the beam is still holding the roof up, another tree will have grown, thus continuing the carbon draw down and storage that the original tree may have maintained. The transformation of biomass into biochar works more or less the same way, by stabilizing the carbon so that it can remain in the ground or in other long-lived material for three hundred or a thousand years. In the meantime, if the used biomass has regrown, the biochar system has increased the terrestrial carbon pool. Even better is the cascading of carbon storage functions, such as building a durable wood house, maintaining it for as long as possible, and when finally it is time to replace the house, or it is destroyed in a hurricane, then to convert it to biochar. Amended into the soil the biochar can increase food and biomass production, while sequestering the C away from the atmosphere for more centuries.
Fig. 7: Cinnamon and Champaca trees (right) and a holy Shiva tree (left), each 1.5 years after planting.
Carbon Accounting of the Forest Garden
The carbon absorbed by a forest garden in Nepal today will eventually return to the atmosphere. But what matters for the climate is the overall accumulation of carbon in the next 10, 40 or 100 years in comparison to the starting situation. In a natural forest the accumulated carbon is contained in all the trunks, branches, leaves, roots, mulch, humus, carbonates, animals, fungi and bacteria. In a new or regenerated forest, this has to be compared with the baseline, e.g. a degraded pasture or field. When a forest is cultivated as a forest garden, construction materials and biochar can be recovered from the forest’s biomass in addition to the harvested food, feed and fiber. This leads to carbon being transferred from a 10– to 50–year average carbon cycle (forest biomass) to a 150–1000 years cycle (construction lumber and biochar). This amount of stored carbon can be added to the accumulated carbon of the forest garden system over the same time period, provided it is harvested sustainably so the trees regrow and the rest of the forest C storage is not impeded or diminished in the long term.
When we evaluate the carbon efficiency of forest gardens, we calculate how much additional carbon is contained within the system (forest garden + stored carbon) in comparison to the previous land use. As long as the forest garden exists, the stored carbon increases the terrestrial pool by this difference. To minimize the risk of significant losses of carbon from the forest garden by inimical events (such as a forest fire), biomass is sustainably harvested and transferred to carbon pools outside the garden, such as construction materials, furniture, biochar, asphalt additives or natural resin. All in all, we anticipate shifting at least 40% of carbon to long-term pools outside of the actual forest garden over the course of 100 years.
Calculation Example for 1 ha of forest garden in Nepal
A hectare of terraced forest garden in the hills of Nepal can support 600 mixed trees accumulating 22t C per hectare in the first 10 years and 70t C per hectare over 25 years in trunk and root wood. During that time, leaves and branches fall to the floor each year and usually rot quickly, nourishing the soil biology. The carbon that is shed with the leaves and branches amounts to more than 100% of the carbon that accumulated in the wood during the same time period (Binkley, D. and Fischer, R., 2013, p.113). Thus, during 25 years, while 70 t of C were accumulated in trunk and root wood, at least 70 t of C were additionally shed in the form of leaves during the same period. If each year a third of the shed or cut forest garden leaves and twigs are collected and pyrolyzed with 30% C efficiency, then 7t C (= 1⁄3 * 70 t C * 30%) could additionally be fixed. Moreover, over 25 years larger branches will have been pruned, or broken off by the wind, and some sick trees will have been taken out of the system as well. Converting this “pruned” wood to materials or biochar, contributes to further carbon storage. New branches and trees will replace the old ones, pulling the same amount of CO2 from the atmosphere. The wood that has been pulled from the system over 25 years can be estimated to account for a third of the total wood produced during this time. If pyrolysed with a carbon efficiency of 30% either directly or after the material end of life, another 7 t C will have been displaced to longer-term carbon pools through the extraction of timber, silk, rope, oil and resin and eventually pyrolysis. We can add the biomass that has been withdrawn and diverted to carbon pools with a long residence time to the total amount of carbon stored in the forest vegetation. Overall, one hectare could absorb at least 84 t C (70 + 7 + 7), or 300 t CO2 from the atmosphere over a 25-year period.
If the forest were to burn down after 25 years, much of the accumulated carbon in the standing forest would immediately return to the atmosphere, however, the roots and some of the trunks would remain intact. In many species, the trunk and/or roots sprout again shortly after the fire. If we assume that 20 t of C persists after the fire and natural regrowth occurs, then the garden has still sequestered 34 t (20 t C + 14 t C that was sequestered in building materials and charcoal in the years prior to the fire).
According to the system established by the Ithaka Institute, only the amount of carbon accumulated in the forest garden through biomass after 10 years (22 t C) - in other words, only one quarter of the carbon expected to accumulate in 25 years (84 t) - is being traded in the form of CO2 certificates. Even if a forest fire were to occur after 25 years (which would normally not happen to more than a small fraction of the gardens), the carbon remaining fixed in the terrestrial pool (34 t C) would be more than the CO2-certified amount (22 t C). The CO2 calculations for forest gardens that have been certified this way are thus very robust, and can be guaranteed with a high degree of certainty. This provides assurance to CO2-subscribers that a one-hectare forest garden will at least accumulate 81 t CO2eq(22 t C) from the atmosphere and effectively sequester it for a long-term within the terrestrial system. Furthermore, it is anticipated that the humus content of the soil will increase, though this has not been integrated into the certificate calculations.
Fig. 8: Thanks to the creation of tree nurseries that provide seedlings for the forest gardens, groups of women are able to earn an additional income. This focus on cultivation of the tree saplings improves the motivation to care for the trees once they are planted out.
In the future, we will be able to estimate the amount of C drawn from the atmosphere more accurately due to a broadening database and growing experience. We can then trade larger amounts with suitable warranties. Presently, however, we prefer a more conservative approach, only trading the CO2 we can vouch for with a high degree of certainty.
Monitoring System
Every certified tree is accurately mapped and dated with a GPS-based smartphone application. At the end of the first and second year, tree height and trunk diameter at 10 cm above ground are measured. After the third year, trunk diameter is measured at chest height (140 cm above the ground), the tree’s general vigor and health are rated on a scale from 1 to 10, and a picture of each tree is taken. This monitoring system serves to ensure that CO2 certificates are issued only for trees that are actually growing well. Customers who bought CO2 certificates will know where “their” trees are, how well they are growing, and how the biomass carbon is eventually sequestered. The goal is that CO2-subscribers will be able to follow online the growth of the forest garden that reclaims their CO2 emissions and they will know which family does the work for them, forming a virtual alliance.
General Work and Project Organization
The most difficult part of such a project is organizing the work among so many families. If we were to employ industrial methods, satellite-guided tractors could dig the tree holes, insert the nutrients and biochar-substrate in the root zone, plant the saplings and spend the rest of the year weeding, fertilizing, watering, replanting and deploying the pesticides that monocultures require. Fast-growing trees like eucalyptus could be planted, row after row, across thousands of square kilometers. If it were only about the carbon, such a system could be quite efficient. As soon as government carbon taxes pay out more than 80 euros per ton of carbon, such a system would become economically viable. Our goal, however, is not only to harvest carbon from the atmosphere but also to establish robust agricultural ecosystems upon which a rural economy can thrive, and to provide long-term employment, income, and a positive outlook on life for young people in remote villages.
Fig. 9: Rows of Champaca trees on a former rice terrace, 18 months after planting.
Our projects are located directly within the villages and on the private land of the farmers. Instead of entering into a 500-hectare contract with a single entrepreneur, we are contracting with 1,000 families who each own about half a hectare. Students of economics learn that such business models are economically inefficient and administrative expenses would eat up profits. However, this is not a purely economic model, but also a socio-ecological management system that provides income for 1,000 families, re-cultivates degraded areas, and establishes water supplies as well as integrating other natural resources. The element of carbon farming, however important, is just one more added benefit.
How can a whole village be motivated to do what is on many accounts probably the right thing but lacks proof of success, especially when the desired success starts showing itself only after two, three years or maybe longer? Trust must be built up first. This can only be achieved by demonstrating the results of such a joint effort.
To build confidence, we first began with simple agricultural trials in each village. With one pilot group per village we manufactured biochar-based fertilizer, demonstrated its use, and established comparative tests. The results of highly improved harvests fostered trust and eventually understanding as to why something works better than what was done before. It also rouses the curiosity of those who did not take part. Personal engagement is essential for such an endeavor and cannot simply be orchestrated from an office through central administration. Joint work creates solidarity, and if successful, the gratitude and joy over something created together is manifest. Few individual families possess financial reserves to afford a pond, a greenhouse for raising plants or a tree nursery by themselves. As a group, however, with modest support provided in the form of seedlings, tools or daily wages these developments became possible.
By cooperating not only with individuals, but also with the village as a whole, we “outsiders” encourage the families in the villages to work together amongst themselves. Of course, in any village not everyone gets along with each other, but when a community works towards a common goal, a new dynamic and solidarity is born. Although this sense of unity remains fragile and has to be constantly tested and renewed, it is the only way to tackle larger community projects such as reforestation, protection of water sources, communal water systems and cooperative product development.
Fig. 10: Ginger has been planted in the half-shade between one-year-old Champaca trees that are used for the manufacture of perfume, fodder and timber.
Our experiences in Nepal have shown that, above all, it is human factors that build a solid foundation towards new paths (or gardens); a purely industrial approach cannot be counted on to achieve the same. Though we might wish for presentable results quickly, we must remain patient. We found we had to constantly learn from our mistakes, rather than entrench them because of fear to change course, due to the sheer size of a project. It is a grave mistake to believe that one is working to save the global climate, while neglecting to ensure that each tree is planted and cared for by a real person in their homeland. This is the difference between our project and many others: We do not wish for monocultures on many-thousand-years-old terraces, or hierarchies based on vested rights; instead, we wished for equal participation of all farmers and families.
Concrete Organization of Planting and Tree Care
One year after the initial forest gardens were planted, the results were quite variable. While some families had kept 95% of their trees alive during the first, most challenging year and replanted the remaining 5% all on their own, others only showed tree survival rates of 30% or lower. Most plantings reached success rates of 60 to 70%. Although that is more than twice as much as in other reforestation projects within the same country, there was certainly much room for improvement.
The main reason behind saplings dying was that the necessary maintenance measures had not been undertaken in a timely way. The irrigation ponds were not refilled in time, and trees had been watered too late. Or the ground had not been sufficiently mulched due to a shortage of readily available leaf matter and a lack of understanding of how important this was. Or the soil had not been piled up and drainage trenches were not built in time before the start of the rainy season. People had hoped that things would work themselves out somehow, and lethargy would prove to be a more efficient way of spending vital energy than over-activity. Even though the families knew they would only receive the CO2 money for their trees if at least 80% survived, not one family knew precisely how many trees on their terraces had survived in the end. None had taken the initiative to count on their own. Therefore, it is our vital task to improve the work organization and to make the incentives and reasons behind various actions clearer.
Establishment of the Triad System
After consulting with experts in the field of psychology, we have established a triad system in which three families form a community that is responsible for the planting of each individual family. Families must communicate the recurrent tasks amongst each other, frequently patrol the plantations of each family, determine failure rates, and replant together. Each member of the triad is responsible for his or her two partners as well. Only if all three families can show a success rate of at least 80% are they entitled to receive the carbon premium. If for example one family reaches 97%, another 83% but the third only 72%, then the triad as a whole has failed and none of the families earns the premium.
If all three partners within the triad surpass the 80% mark, each one of them receives the carbon bonus in accordance with the number of trees that are growing strongly on their own terraces. However, they have committed within the triad to regrow the missing trees together. The project provides 100% of the seedlings necessary for replanting if a triad has at least an 80% survival rate. Triads that have not reached their 80% have to pay for the new seeds themselves, but after successful replanting they will get paid the carbon premium for the number of trees that survived. The intent of this system is not to distribute gifts, but rather to encourage accountability, promote self-responsibility and most of all personal initiative.
Multiplication of the Reforestation
Another aspect of triad organization is that from the second year on, each family in a triad must become a mentor of a new triad in another village and supports them in their self-organization. We acknowledge that this could turn out to be difficult, since not everyone is suited to become an organizational sponsor—to say nothing of the tension between villages. Nevertheless, it seems to be the most promising method to spread this kind of reforestation from village to village and all across the region.
Fig. 11: Intercropping of cinnamon, mulberry and Champaca trees. Hari Maya, the farmer in the picture, is 76 years old and filled with pride and joy from being able to witness this new awakening in her village. To set an example for the younger generations, she has single-handedly planted 360 trees on her fallow terraces.
The Ithaka Institute does not aim to organize and outsource the reforestation and connect forest gardens to the global CO2 market in the form of a continuously growing commercial business. Our goal is to develop models and demonstrate their practical implementation, so that they will be emulated and improved by as many other people as possible. We hope these models will open doors for the development and implementation of many similar projects. To be successful in effectively slowing down climate change over the next 20 years, we need millions of these kinds of forest garden projects. We regard it as our duty to not just design various successful models but to put them into practice as an example for others to replicate and improve. Only through practical implementation can we discover and fix any weaknesses and flaws in the system. By dealing with such complications in an open manner, we can offer recommendations for action and establish eco-social methods that create opportunities to reverse climate change.
It is our outspoken and immodest goal to develop the CO2 Forest Garden Systems with such transparency and success that they will be emulated, improved and adapted to specific cultural and ecological conditions in many different countries and regions. Between Istanbul and Shanghai there are millions of square kilometers of deforested area, just as there are across the Mediterranean region, in large parts of Africa, Australia, Mexico, Iceland and the southern part of the USA. Converting depleted landscapes to productive forest garden systems holds the potential to change the way we manage our planet’s resources for the better, and to establish a global management of the carbon cycle (global carbon governance).
Current State
Since the summer of 2015, over 36,500 trees have been planted in cooperation with 89 farming families; 49 ponds have been put into place; and five villages are now housing tree nurseries. While only 60% of the planted trees survived the first year and 60 tons of CO2 have been extracted from the atmosphere, the survival rate for the second year reached 77.5%. The introduction of triads improved the success of the plantings, but surprisingly only 8 out of 29 triads succeeded in receiving the carbon bonus at the end of the year. However, seven out of the eight triads that succeeded had participated already in the first year; experience, apparently, played an important role both for the cultivation of the forest garden as well as for the social interaction within the triad.
A good number of trees from the first plantation campaign have already grown so much that the first harvest feels almost palpable. The visible excitement of the farmers over witnessing the fruits of their labor and how the landscape has changed over such a short time span is our greatest reward, and also serves to motivate us toward new steps and trials and to promulgate our findings.
While the necessary investments in seeds, planting, water retention, watering, etc. would not have been possible for the farmers without CO2 certificates, we are now anticipating that the yield from the trees after just five years is going to be fifteen times as high as the certificates themselves. The trees will continue to draw CO2 from the atmosphere, but the farmers will no longer depend on global trade for additional income. That does not mean that they will no longer be rewarded for their services to the global climate, just that they will not have to depend on it anymore.
ReferencesBinkley, D and Fisher R. (2013): Ecology and Management of Forest Soils. Wiley-Blackwell edition, Oxford, UK.
Boysen LR, Lucht W, Schellnhuber HJ, Gerten D, Heck V, Lenton TM. 2016. Earth ’ s Future The limits to global-warming mitigation by terrestrial carbon removal Earth ’ s Future. 1–12. DOI: 10.1002/eft2.203
European Environment Agency greenhouse gas data viewer (2017): http://www.eea.europa.eu/data-and-maps/data/data-viewers/greenhouse-gase..., retrieved on 15th March 2017.
Hansen J, Sato M, Kharecha P, Schuckmann K Von, David J, Cao J, Marcott S, Masson-delmotte V, Prather MJ, Rohling EJ, Shakun J, Smith P. 2016. Young People ’ s Burden : Requirement of Negative CO 2 Emissions. 1–40. DOI: 10.5194/esd-2016-42
Lehmann J, Abiven S, Kleber M, Pan G, Singh BP, Sohi SP, Zimmerman AR. 2015. Persistence of biochar in soil. In: Lehmann J and Joseph SD (eds) Biochar for environmental management. Routledge: London, 235–299
Rockström J, Gaffney O, Rogelj J, Meinshausen M, Nakicenovic N, Schellnhuber HJ. 2017. A roadmap for rapid decarbonization. Science 355
Rockström J, Schellnhuber HJ, Hoskins B, Ramanathan V, Schlosser P, Brasseur GP, Gaffney O, Nobre C, Meinshausen M, Rogelj J, Lucht W. 2016. The world’s biggest gamble. Earth’s Future 4: 465–470. DOI: 10.1002/2016EF000392
Rockström J, Steffen W, Noone K, Persson Å, Chapin FS, Lambin EF, Lenton TM, Scheffer M, Folke C, Schellnhuber HJ, Nykvist B, de Wit CA, Hughes T, van der Leeuw S, Rodhe H, Sörlin S, Snyder PK, Costanza R, Svedin U, Falkenmark M, Karlberg L, Corell RW, Fabry VJ, Hansen J, Walker B, Liverman D, Richardson K, Crutzen P, Foley JA. 2009. A safe operating space for humanity. Nature 461: 472–475. DOI: 10.1038/461472a
Toensmeier. (2016). The Carbon Farming Solution (Chelsea Gr). White River Junction.
Umwelt Bundesamt (UBA, 2017): CO2-Rechner. http://uba.co2-rechner.de, retrieved on 15th March 2017.
World Bank/Ecofys/Vivid Economics (2016): State and Trends of Carbon Pricing 2016. Washington, DC: World Bank. © World Bank https://openknowledge.worldbank.org/handle/10986/25160. License: CC BY 3.0 IGO.
Zimmerman AR, Gao B. 2013. The Stability of Biochar in the Environment. In: Ladygina N and Rineau F (eds) Biochar and Soil Biota, 1–40
Fig. 12: Paulownia trees are among the three fastest growing types of trees. This Paulownia was planted in a mix of compost and urine-impregnated charcoal. It has reached 4.85 meters in 9 months.